
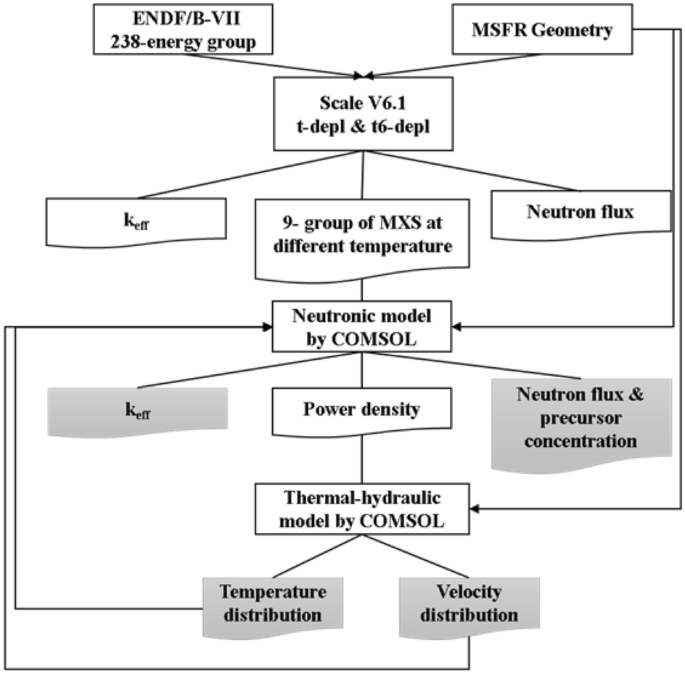
The sound pressure level above the liner is computed and can be compared to results from a published research paper. The liner consists of eight resonators with thin slits and the background grazing flow is at Mach number 0.3.
#COMSOL 5.3 STOKES HOW TO#
This model demonstrates how to compute the acoustic properties of an acoustic liner with a grazing flow. The heavier particles are expelled radially outwards due to the centrifugal force and exit together with a small fraction of the flow through the lower outlet.Īpplication Library path: CFD_Module/Single-Phase_Tutorials/hydrocyclone New Tutorial Model: Acoustic Liner with a Grazing Background Flow The lighter particles are entrained in the flow and follow the main flow upwards to the upper outlet. All fluid-structure interaction models in the Application Libraries have been updated to include this new coupling functionality.įlow and particle trajectories in a hydrocyclone.
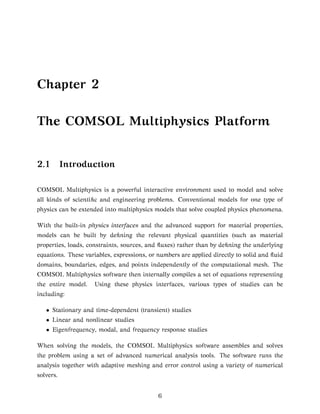
After adding a Fluid-Structure Interaction interface from the Model Wizard, you will get a Solid Mechanics interface, a Laminar Flow interface, a Fluid-Structure Interaction multiphysics coupling node, and a Moving Mesh node in the Definitions section. On the fluid side, all turbulence models are now available as well as a number of new boundary conditions. On the structural side, many additional boundary conditions and material models are now available for FSI analysis for example, rigid domain, piezoelectric, and nonlinear elastic material models. With this approach, all functionality in the constituent physics interfaces is available for fluid-structure interaction (FSI) modeling. The new coupling matches the modern style, with a number of single-physics interfaces and multiphysics nodes to couple them together. New Fluid-Structure Interaction Interface That Supports All Turbulence ModelsĪ new Fluid-Structure Interaction multiphysics coupling has replaced the interface used in previous versions of the COMSOL ® software. The rotating machinery interface is also available for all turbulence models for more vigorously stirred mixers and tank reactors. The tutorial model of a gently mixed liquid solution utilizes the new rotating machinery interface for laminar flow. All the applicable models in the Application Libraries have been updated to take advantage of this boundary condition. Overall, this leads to more accurate and realistic physical models. Additionally, it does not constrain the temperature at the inlet's adjacent edges (or points in 2D), but instead assigns a heat flux that is consistent with the upstream conditions. Applied at inlets, where you would previously apply a Temperature boundary condition, the Inflow condition accounts for temperature and pressure from upstream phenomena. The new Inflow boundary condition applies an inflow of heat coming from a virtual domain, which has been excluded from the model to simplify the analysis, with known upstream conditions. Finally, simulations are augmented to account for the influence of bath potential and approaching a charged surface on pipette dynamics.The inlet from a nozzle with star-shaped cross section is modeled using the fully developed turbulent flow inlet condition. Moreover, the pipette tip potential is accurately modeled as a function of pipette radius and cone angle, enabling deduction of geometric properties from easily measurable tip offsets. Thus, users can precisely understand how electrical constraints propagate through to the pipette-cell interface. The simulations replicate the typical experiment of a nanoscale pipette entering a neuron, and are able to calculate pipette resistance and efflux, as well as ion concentration profiles, for current- and voltage-clamping situations.
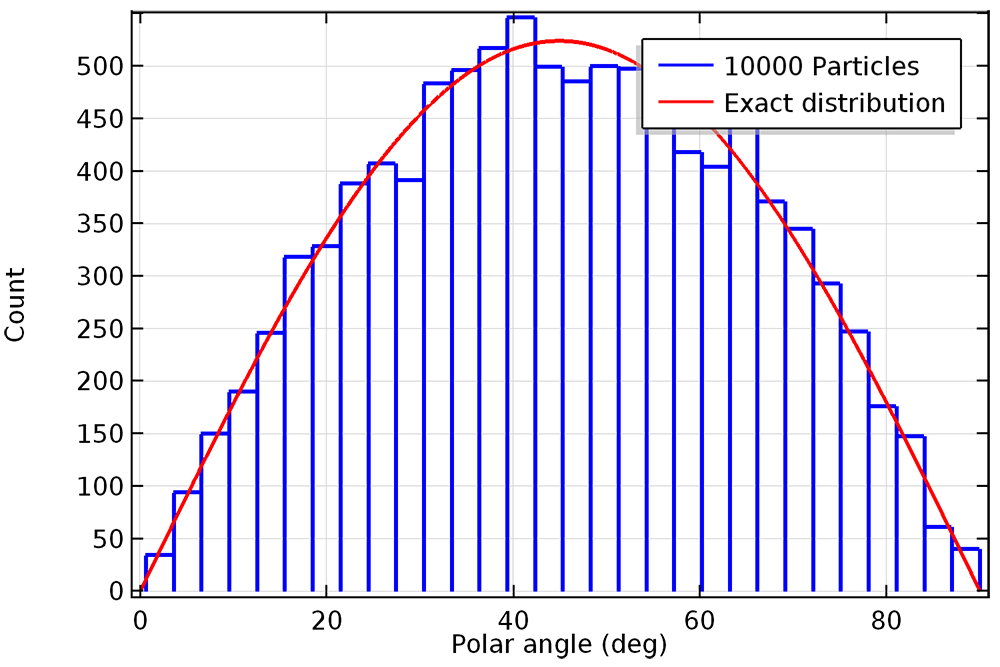
The present work simultaneously solves the Poisson, Nernst-Planck, and Navier-Stokes equations using the electrostatics, transport of diluted species, and laminar flow physics modules in COMSOL 5.3 software. In doing so, experiments with such devices can be more finely tuned to influence neurons as intended, and geometric device parameters can be inferred through simple system measurements. Numerical modeling offers a solution to both drawbacks, as finite element simulations can accurately predict the electrodynamic properties of nanopipettes. Moreover, nanoscale pipettes are difficult to reproducibly manufacture with high precision, and time-consuming to acutely characterize. However, bulk electrodynamic properties begin to break down at nanoscale openings, introducing unusual effects that must be understood in order to properly evaluate experimental data.

Nanoscale pipettes allow for exciting extensions of traditional current- and voltage-clamping electrophysiology experiments.
